Chapter 11
What we found: Exercise and muscle performance
What you’ll learn in this chapter
In this chapter, we’ll look at what genetic testing can tell us about:
- how we might respond to or recover from exercise; and
- whether we can predict our athletic performance or talents from the makeup of our muscle fibers.
Two important points to keep in mind:
- While science is cool, and we have some interesting genetic findings and areas for further exploration, we still know comparatively very little.
- Just because a genetic test can tell you (for instance) what kinds of muscle fiber types you might have, it doesn’t mean that it can tell you the “perfect” exercise plan for you.
As you read this chapter, remember our usual caution:
As with most preferences, health risks, and genetic traits, there are many complex, interrelated factors.
There is almost never one single gene that inevitably leads to a given result.
Any genetic data we share are simply clues for further exploration.
What determines our physical capacity?
Maybe you’ve looked out your window early one morning, watched the dedicated runners glide past, sleek and lean, and thought, “That will never be me.”
Maybe — likely around December 31 each year — you’ve thought, “I should take up running”. Then you abandoned it around January 12 when your knee said, “Bad idea. Smarten up next year.”
Maybe you are a runner, and wondering how you could be better.
Maybe your personal trainer is telling you that sprinting is awesome, but all you want to do is chill out with an easy trail run. Or the other way around — every time you try to run more than 5 minutes, you want to lie down until the world stops spinning.
Maybe you’ve already run 3 miles in the time it took us to read this.
Have you ever wondered whether you are “naturally” meant to run (as Christopher McDougall suggests in his book Born to Run)?
Or to do any other type of sport?
It’s a good question, one without clear answers. (Yet.)
Human physical capacity is complex.
Human movement is complex.
There are no known “golf genes”, “parasailing genes” or “hip-hop dance genes”.
Yet genetic data can give us some clues about how we might play to our potential.
Are you a tortoise or a hare?
Sprint versus endurance performance
You might have seen some version of a photo series floating around the Internet that compares a sprinter’s muscular, powerful body to an endurance athlete’s lean, sinewy one.
The usual implication is that if you want to look like a sprinter, train like one.
Indeed, fitness media is full of workout programs that promise you the body you’re seeking:
- Want “long, lean muscles”? Do Pilates or yoga.
- Want to be tall, slim, and graceful? Do ballet.
- Want to be jacked and lean? Do Crossfit.
- Want to be a human tank? Do rugby.
And so on.
Is it that simple? Nope.
Of course, nobody is “genetically gifted” enough to just roll out of bed and into an Olympic-caliber performance or a magazine-cover-worthy physique. In other words, training and nutrition matter… a lot.
Certain types of training do, indeed, amplify physical abilities and characteristics such as muscle size or mobility. So you could probably become more graceful after years of ballet classes, more muscular after years of bodybuilding, or more likely to grind other people’s faces into the mud after years of rugby.
Yet athletically “ideal bodies” — in other words, bodies that demonstrate elite, world-class performance and physique development — involve a statistically unusual collection of physical characteristics that are, for the most part, present on Day 1 of training.
In other words, as Lady Gaga sings, genetically speaking, these top athletes were born this way.
This is particularly true with events at the polar ends of the running continuum (e.g., 100-meter sprints compared to endurance or ultra-endurance), or the size continuum (e.g. gymnastics and horse racing versus basketball and sumo wrestling).
Yet other events that include sprint or endurance performance also include other elements, such as hand-eye coordination and reaction speed. Our co-contributor John, a highly-ranked master’s-level sprinter, reports that although he could easily match NFL skill position players in a 40-meter sprint, he got “obliterated” when competing in a reaction time contest against them.
Although most youth coaches know intuitively that some athletes show up with better physical raw material than others, it’s hard to say exactly what the genetic basis of those gifts might be.
What parts of athletic performance come from our so-called “genetic potential”?
And what parts are a result of training and practice?
Exercise physiologists have suggested that many factors could contribute to running performance in particular, such as:
- Creatine kinase (CK), an enzyme involved in cellular energy production cycles and in passively moving this energy from mitochondria to myofibrils (muscle fibers) in contracting muscle. Some studies suggest that having the AA version of the rs8111989 SNP in the CK-MM gene, which codes for CK, may improve physical performance in various tasks. CK-MM polymorphisms have also been linked to differences in muscle damage after exercise.
- Maximal oxygen uptake, aka VO2 max, which may be responsible for the better endurance performance of athletes from mountain populations, such as the East African highlands, the South American Andes or northern Mexico’s Sierra Madres, home of the famed indigenous Rarámuri / Tarahumara runners. Although we can improve VO2 max a fair bit with training, there’s also a significant genetic contribution: researchers speculate that between 40-50% of variance in oxygen uptake is genetically determined.
- Tendon stiffness, particularly in the lower leg, which could offer more elastic “spring” to a stride.
- Skeletal structure, including narrower hips.
- Mitochondrial gene expression, mitochondrial DNA and mitochondrial enzyme activity: Since mitochondria are the “power generators” of a cell, better mitochondrial function may mean more sustained energy for athletic activity.
- PGC-1α is a protein that binds to and activates transcription factors, including most nuclear receptors. It’s strongly expressed in skeletal muscle, particularly Type I oxidative fibers. Transgenic mice with more PGC-1α have improved endurance performance and preserve muscle mass better, particularly as they age. (More on muscle types below.) In humans, PGC-1α increases when we exercise, and may coordinate the activation of metabolic genes in muscle in response to exercise. It may also improve the function of mitochondria and peroxisomes, which break down fatty acids.
These are a mix of environmental and heritable factors.
Even something that may seem as “fixed” as skeletal structure can be affected by our environment.
For instance, let’s say we have genetically-identical twins separated at birth.
- One grew up in an affluent region with plenty of good nutrition and early-life sports training.
- The other grew up in a poor region with frequent food deprivation and malnutrition, where “sports training” was kicking an old soccer ball around and walking miles to get fresh water.
Although there will obviously be a family resemblance, the second twin will probably end up with a somewhat different build — likely lighter and smaller than the first twin.
There’s no single “sprinter gene” or “marathoner gene”.
At last count, researchers have found more than 200 genetic variations that may contribute to physical performance, or how well people respond to training.
Even capacities that may seem fairly simple, like “endurance”, are actually complex abilities that depend on many factors.
For instance, among endurance athletes from East Africa, how much do cultural factors contribute to developing endurance capacity and performance — for example, is there a “running culture” where children are encouraged and supported to run early in life? Do strong runners have a high social status?
What about the diverse geography of countries like Ethiopia and Kenya, which contain both highlands and lowlands — is there an “oxygen advantage” for athletes who grow up at higher altitudes?
Similarly, Chris McDougall’s book Born to Run looks at the Rarámuri / Tarahumara population, who have physiological features that make them good runners, but who also live in mountainous regions where running is a favored activity.
Do athletes from these isolated regions have distinct genotypes?
Or have they simply adapted their fitness and behavior to match to lower-oxygen environments and/or “movement cultures”?
The Spanish ultra-athlete Kilian Jornet is an example of why it’s hard to answer such questions.
Jornet specializes in FKTs, or “fastest known times” for ultra-endurance activities — for instance, he set the record for a round trip up Mount Kilimanjaro in 2010 (7 hours 14 minutes). His VO2 max is around 85-90 mL per kg of body weight per minute, compared to the average fit male’s VO2 max of between 45-55.
This sounds like he’s a genetic freak designed for endurance work, and maybe he is… but he also grew up as the son of a mountain guide in the Spanish Pyrenees, in a rustic mountain hut at an altitude of about 6,500 ft (2,000 m).
So what is responsible for his performance?
- His genes?
- His lifetime physiological adaptation to living at high altitude?
- His upbringing?
- His family environment?
- His training?
Of course, the answer is probably “All of the above, and more.”
One study looked at genetic polymorphisms that may be involved in endurance performance. Using 46 world-class endurance athletes and 123 controls (all white Europeans in Spain), this study set out to explore whether there was an “ideal endurance type”.
Researchers tested the subjects for the following seven genetic variants, involving many factors that are part of endurance performance:
- The insertion / deletion variant of ACE, which codes for angiotensin-producing enzyme (ACE). ACE helps control blood pressure and inflammation. The relationship between variants in this gene and endurance performance were first noticed in high-altitude British mountaineers who were able to climb higher than 7,000 meters without using supplementary oxygen.
- The Arg577Ter variant of ACTN3, which codes for alpha-actinin-3, and which we’ll look more at below.
- AMPD1 Gln12Ter, which codes for an enzyme that deaminates AMP (adenosine monophosphate) to IMP (inosine monophosphate); variations can lead to impaired exercise performance or myopathy (damage to or diseases of muscle tissue).
- Muscle-specific creatine kinase (CKMM 1170 bp/985 + 185 bp variant), a genetic variant associated with aerobic performance.
- HFE His63Asp mutation; HFE codes for the human hemochromatosis protein and is involved in iron uptake.
- GDF-8 Lys153Arg mutation, aka MSTN, which codes for myostatin, a major determinant of how much muscle mass we can have.
- PPAR-𝞬 (PPARGC1A Gly482Ser), related to genes involved in energy metabolism, muscle fiber type, blood pressure, cholesterol metabolism, and obesity.
Would endurance athletes likely have more of the particular variations that promote athletic performance than average? Yes.
But only three of the 46 top world-class endurance athletes had the best possible score for up to six genes.
More significantly, none of them had the “perfect profile” of genetic variations.
Other researchers took this speculation one step further, asking:
Could the “perfect” endurance athlete (at least based on what we currently know about genetic polymorphisms that favor endurance) theoretically exist?
These researchers put together 23 polymorphisms that were strong contenders for individually influencing endurance, and considered how likely it was that this “perfect endurance athlete” could exist. They speculated that among people of white European backgrounds, only 0.0005% of them might have this profile.
In the United States, there are around 224 million people who report their main ethnicity as white European. This means that using this model, 1,120 people might have this genetic profile.
Should those 1,120 people report for marathon training immediately? Well, even if we could find them, lots of other factors might affect their performance, such as:
- Whether they actually like endurance exercise;
- Whether they are motivated to train;
- Whether they have the mental skills to stay focused during long events;
- Whether they want to spend hours pounding the pavement, or would rather watch Netflix;
- Whether they live near somewhere they can train;
- Whether people around them are doing the same activity;
- Etc.
Some researchers suggest that among top athletes, about two-thirds of their athletic capacity can be explained by genetic factors that add up, with the remaining one-third being explained by environmental factors.
Yet that theoretically “perfect profile” may also depend on an athlete’s ancestry.
In a study that compared white European and East Asian swimmers, top swimmers did have the ACE Ins/Del variation, but top Europeans tended to have the D allele, while the top East Asians tended to have the I version (ACTN3 was also tested, and it didn’t seem to make a difference).
What this means for you
- It’s complicated. (Darn it.) Most sports and physical activities involve a mix of capacities, only some of which are strongly genetically influenced.
- While we might be able to predict which people have the highest ceiling for athletic development, we can’t predict if a person will ever hit that ceiling. We have some compelling clues and strong hypotheses, but not enough data yet to build a model.
- Most coaches will probably tell you that they would get better data from simply knowing, observing, and understanding their athletes than from genetic testing. Standing in a field with a clipboard, watching an athlete train for several months or years, is probably the best data of all.
- Heredity is not destiny. Even if you come from a population of people that have traditionally done well at a certain activity, it’s no guarantee that you will do the same, or that you have any intrinsic “natural ability” by virtue of heredity. Conversely, even if you come from a population of people that traditionally haven’t done well at a certain activity, it’s no guarantee that you can’t succeed at that activity.
The genetic makeup of muscle fibers
Muscles are made up of fibers. Within each muscle fiber are many myofibrils, bundles of long polymers of the proteins myosin and actin. When myosin and actin filaments slide past each other, we get muscular contraction.
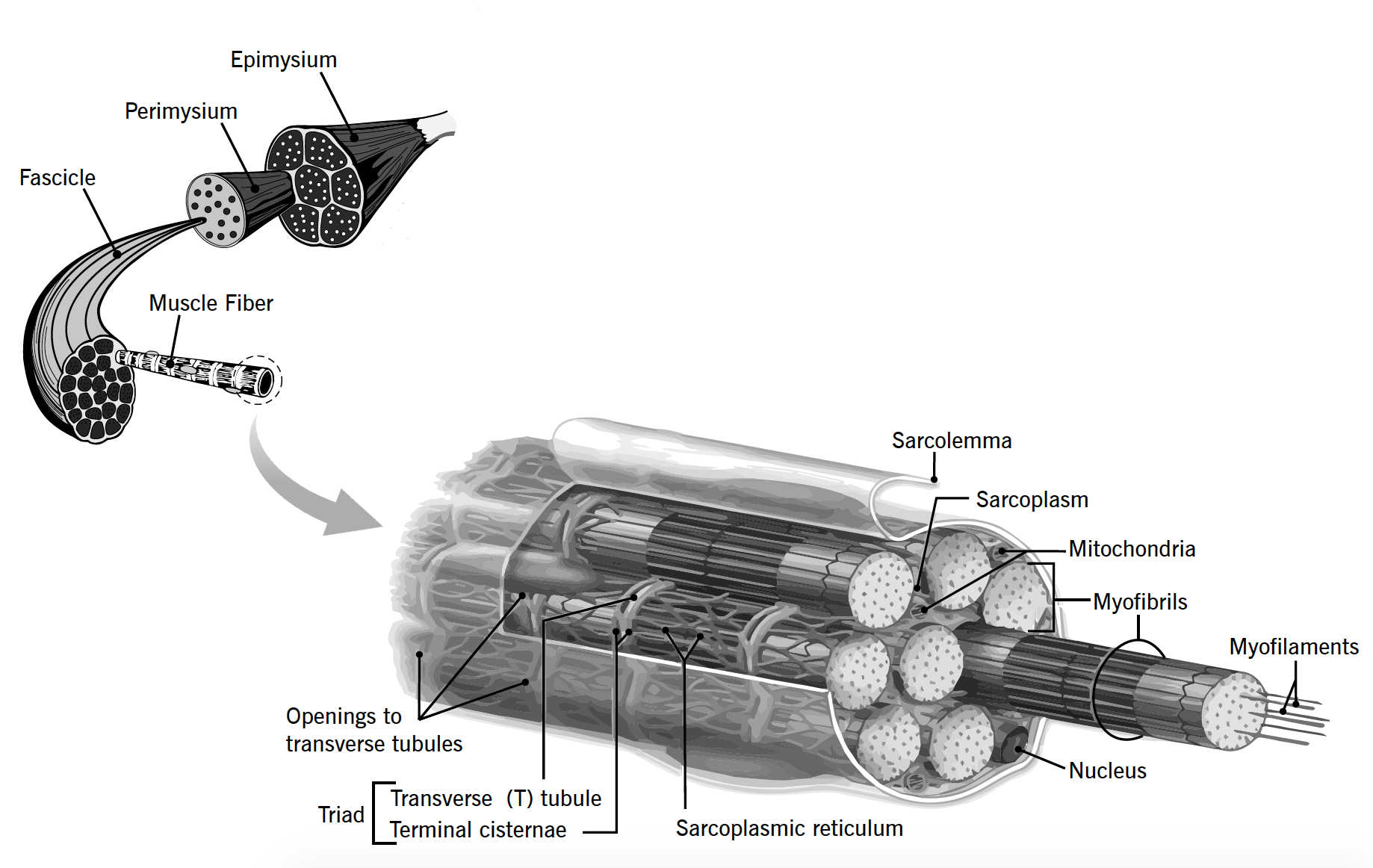
Actin is an ancient protein that has long been conserved through evolution. In mammals, there are six actin paralogs: different yet related forms encoded by separate genes. One study describes actin as “cellular steel”, because actin can act as an “alloy” to form various mixes of protein-based structures in cells.
There are likewise many classes of myosin proteins; myosin II is the type involved in muscular contraction. Nearly 20 known genes contribute to myosin II.
ACTN3
The ACTN3 gene makes a protein called alpha-actinin-3, which (unlike its buddy ACTN2) is only expressed in fast-twitch muscle fibers, which we use for speed and power movements like weightlifting, sprinting, and/or jumping.
Research suggests that particular forms of this gene correlate to sprint and endurance performance. 23andMe looks at a particular SNP (rs1815739) on the ACTN3 gene.
The T form of the rs1815739 SNP prevents the full alpha-actinin-3 protein from being made, and people with two copies of T lack alpha-actinin-3 completely. Thus, many elite sprinters and strength athletes have the CC type, while few have the TT type. CT is a mixed type.
Among athletes, power athletes were much more likely to have at least one working copy of the gene than non-athletes, and at elite levels, nearly everyone has at least one working copy (in other words, they were either CC or CT).
Seems pretty straightforward: If you have the right ACTN3 variant, you should be crushing all the strength-power events, right?
As it turns out, so far, only running seems to be strongly affected. It doesn’t seem to make a difference for other movements like throwing and jumping. Nor does it seem to matter for team sports.
This doesn’t necessarily mean that TT makes you a better endurance athlete (since research suggests that being a TT gives elite cyclists no advantage), but rather that if you’re a TT, you may perform less well in sprint and power-type events.
On the plus side, while TTs started out weaker in workout programs, they often made significant gains when trained.
Nor does a TT type seem to cause any type of disease. This may be because there are other related proteins that can do the job, though less well. For instance, ACTN2, which is expressed in all muscle fibers, might compensate for the loss of ACTN3 in fast Type II fibers.
Alpha-actinin-3 may also affect how muscles use oxygen. Research suggests that having less ACTN3 might make muscles greedier for oxygen, which might be metabolically costly and slow the CT or TT people down. Studies in mice have found that muscle fibers lacking alpha-actinin 3 are weaker and smaller, but more efficient and fatigue-tolerant — a perfect recipe for an endurance athlete.
What we found in our sample
- About one-third of us have the “pure fast-twitch” or “sprinter” CC type of ACTN3 SNP variation
- About one-sixth of us have the TT, or “endurance-type”, variation.
- The rest of us are CT, or mixed.
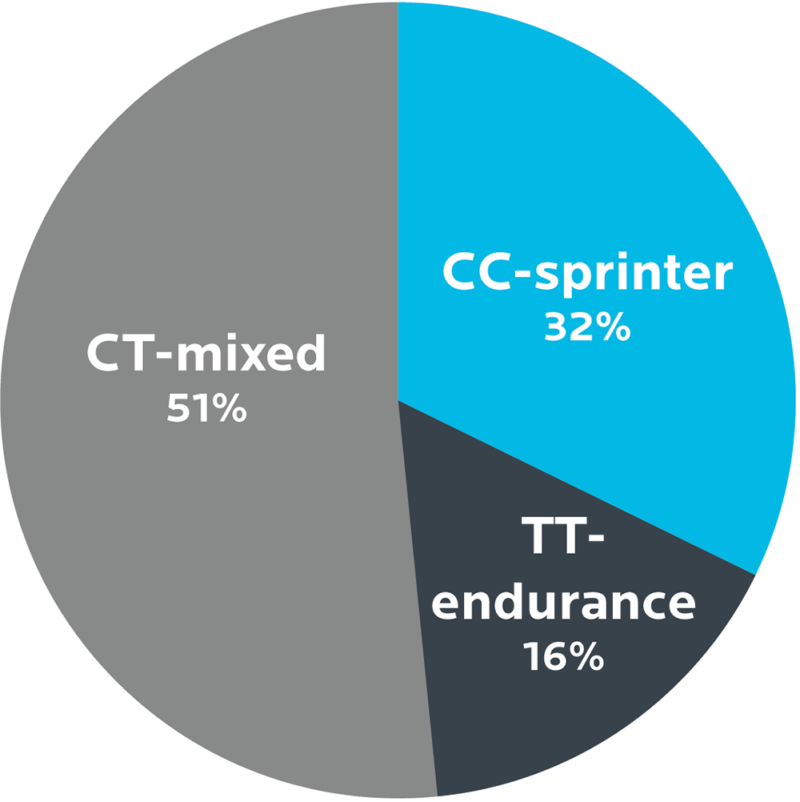
How does this compare to real-world experience?
Among the people we surveyed:
- About half of them matched their expected genetic profile, i.e.:
- CCs preferred sprint-type activities.
- TTs preferred long slow endurance-type activities.
- CTs preferred a mix.
- A little less than half of them sort of matched their profile — in other words, they were CTs who preferred either sprint or endurance-type activities. We counted this as a partial match, since in terms of their muscle fiber makeup, CTs could likely go either way.
- 5% of them were the opposite: either CC hares who nevertheless preferred endurance-type activities, or TT tortoises who nevertheless preferred sprinting-type activities.
Our PN sample is a handy one for this particular SNP, because it contains many people who have achieved significant athletic success (i.e., who were competitive at the national or international level).
Interestingly, there was no clear correlation between a given SNP combination and a predicted “match” for a given sport.
In fact, this high-achieving athlete group included someone with the “opposite” SNP to what they “should” have been doing.
What this means for you
- Genetic testing can tell you what form of the ACTN3 variant you have.
- Both 23andMe and Nutrigenomix test for the rs1815739 SNP in the ACTN3 gene.
- You’ll likely notice a difference in your sprint versus endurance preferences and abilities if you have a “pure” form of the ACTN3 variant — in other words, either a CC (sprinter type) or TT (endurance type). If you’re a mixed type, you might notice you could go either way.
- If you like particular activities but aren’t “genetically destined” to do them, enjoy them anyway. You probably won’t get to the Olympics (though based on our sample, who knows?), but if you’re a CC who appreciates a leisurely Sunday morning jog, have fun.
How well can you recover from exercise?
Being good at movement, sports, and exercise isn’t just about how well you perform during those activities. It’s also about how well you recover afterwards.
After all, it’s hard to become a world-class athlete if most of your training time is spent sitting on an ice pack.
When we move with vigor, we do minor damage that must be repaired, and we put stress on our structures, which then remodel themselves to manage the strain. It’s this repair and remodeling process that makes us stronger and fitter, not the workout itself.
Non-genetic factors in recovery
Age and life stressors play a major role in recovery. You simply can’t recover as well at 81 as you did at 18, nor will you be able to sustain a tough training load if you’re also working full-time as an emergency room doctor with a newborn baby at home.
Biological sex is also a factor, though this is usually hormonal rather than genetic per se (in other words, these are not necessarily characteristics linked to X or Y chromosomes).
For instance:
- Women tend to have more tissue laxity than men (which may mean more joint injuries) as well as a higher rate of many autoimmune diseases (which are typically aggravated by stress, including training stress).
- Men’s higher average testosterone, which helps with protein synthesis, means that they build more muscle faster.
Then there are emotional, social, and cultural components.
For instance:
- Are you a perfectionist, type-A, “second place is first loser” kind of thinker who would rather tango with an alligator than miss a workout? (Or maybe tangoing with the alligator is your workout?)
- Do you choose sports and activities that push your limits? Or are you more of a “chill out with a restorative yoga class” kind of person?
- Do you train on a team where the motto is “Pain is weakness leaving the body” or “Tape it up and get back in there, ya baby”?
- Did you grow up doing manual labor from an early age, and/or in a family where sports, exercise, and movement were encouraged? (In other words, did you start building titanium tendons as a toddler?)
Genetic factors in recovery
And, of course, many factors in recovery are shaped by our genetic expression.
For instance:
- How fast and effectively can your body make connective tissue proteins to repair damage in structures like ligaments, tendons, and cartilage?
- How fast and effectively can your muscles clear waste products and repair themselves?
- How fast and effectively does your immune system respond to the stress of exercise? (Or does it over-respond and start attacking healthy tissues?)
- How does your body manage inflammation? Is it a constantly raging forest fire? Or a carefully controlled tactical operation, complete with fast and efficient cleanup crew?
Given all the factors involved in recovery, there are naturally many potential genetic contributors. Here are just a few.
We won’t look at all of these in depth. Just get the main ideas:
- It’s complex.
- We still have a lot to learn about what genetic factors affect our recovery from exercise.
Genes and variations associated with recovery
Gene / variation | What it’s related to |
Angiotensin gene
ACE (I/D) (rs4646994) |
Creatine kinase and recovery from eccentric muscle lengthening |
Actin protein gene
ACTN3 (rs1815739) |
See above for more on ACTN3; some forms may predispose people to exercise-induced rhabdomyolysis (excessive muscle tissue breakdown) |
Chemokine (cell signaling) ligand and receptor genes
CCL2 −3441(C>T) (rs3917878) CCL2 −289 (G>C) (rs2857656) CCR2 −941(A>C) (rs3918358) CCR2 4439 (T>C) (rs1799865) |
Markers of exercise-induced skeletal muscle damage; soft tissue injuries |
Collagen repair genes
COL1A1 rs1800012, COL5A1 rs12722, rs3196378 BstUI RFLP COL27A1 rs4143245, rs1249744, rs753085, rs946053 TIMP2 rs4789932 TNC |
Remodeling of connective tissues; variants (especially in COL5A1) are associated with higher rates of anterior cruciate knee ligament injury, tennis elbow, carpal tunnel and Achillles tendon tears |
Creatine kinase gene
CKM Ncol (A>G) (rs1803285) |
Creatine kinase as well as C-reactive protein (CRP), a marker of inflammation |
Insulin-like growth factor genes
IGF-II (C13790G, rs3213221) IGF-II (ApaI, G17200A, rs680) IGF-II antisense (IGF2AS) (G11711T, rs7924316) IGF binding protein gene IGFBP-3 (−C1592A, rs2132570) |
Associated with muscle damage (particularly in men) |
Interleukin genes
IL1B −3737 (C>T) (rs4848306) IL1B −511 (C>T) (rs16944) IL1B 3954 (C>T) (rs1143634) IL6 −174 (G>C) (rs1800795) |
Inflammatory response to exercise and muscle damage |
Insulin gene
INS 1045 (C>G) (rs3842748) |
Codes for the insulin protein, which transports nutrients into cells |
Myosin light chain kinase genes
MLCK 49 (C>T) (rs2700352) MLCK 37885 (C>A) (rs28497577) |
Myosin muscle protein phosphorylation; may affect how well muscle fibers can tolerate mechanical force |
Osteopontin promoter gene
OPN −66 (T>G) (rs28357094) |
Muscle size and weakness; muscle damage marker |
Solute carrier family 30 (zinc transporter) gene
SLC30A8 (C>T) (rs13266634) |
Zinc transport and insulin secretion; associated with recovery |
Superoxide dismutase 2 gene
SOD2 (C>T) (rs4880) |
Recovery from oxidative stress |
Tumor necrosis factor gene
TNF −308 (G>A) (rs1800629) |
Creatine kinase (CK) response to eccentric exercise; also regulates muscles’ ability to repair and grow |
COL and TNC genes
One interesting genetic contribution to recovery is our ability to regenerate collagen proteins, which make up much of our tendons, ligaments, and other connective tissues.
The gene COL5A1 codes for the alpha-1 chain of type V collagen.
Variations of this gene have been linked to connective tissue disorders such as osteogenesis imperfecta (aka brittle bone disease) or Ehlers-Danlos syndromes, a group of connective tissue disorders that can range from mild joint laxity to potentially fatal complications if the connective tissues of major organs are affected.
The rs12722 SNP on the COL5A1 gene has been linked to chronic connective tissue injuries such as Achilles tendinopathy.
COL5A1 gene variants also seem to affect the mechanical properties of collagenous tissues, such as its stiffness during moderate to intense contractions (for instance, running, jumping, or other explosive movements).
For many sports, having stiffer tendons and ligaments is an advantage, as these tissues can bear more load and produce more elastic energy to help generate force. Conversely, “looser” joints with laxer tissues may be more prone to injuries, as the tendons and ligaments are less able to maintain stability around the joint.
Similarly, variations in the COL1A1 gene, which codes for collagen type I, are associated with several complex connective tissue disorders, as well as shoulder dislocations and ruptures of the anterior cruciate knee ligament (ACL) and/or Achilles tendon.
Tenascin-C, encoded by the TNC gene, is a glycoprotein that is also involved in wound healing as well as formation of tendons, ligaments, cartilage, and bone. Like variations in the COL genes, variations in the TNC gene are linked to tendon injuries.
What we found in our sample
We didn’t test for any recovery-related genetic variants such as the COL genes, but we did ask people about their experiences of joint injury.
There were clear variations in people’s experiences: Some people felt like they were always injured; others said they almost never had problems despite regular and rigorous workouts.
In addition, only one person who’d been a high-achieving athlete (but started athletics later in adulthood, rather than in childhood, as most others had) reported frequent joint injuries. Most other athletes in the sample said they rarely suffered from joint pain or injuries (or only had problems if they were really pushing their training hard).
Does this mean that athletes “naturally” have better recovery?
Or perhaps that people who started athletics young had better-conditioned connective tissue?
In our sample, it’s hard to say whether there’s any definite connection, but one possible hypothesis could indeed be that to succeed in athletics, you need to survive the training, and this includes joints as well as muscles.
What this means for you
- Genetic testing services may be able to tell you about some of the gene variants linked to recovery from exercise. If you’re considering using genetic testing to explore this question, try to find a service that offers as broad an analysis as possible, rather than just one or two exercise-related SNPs.
- Even if you don’t know your genetic makeup, or don’t have genetic variants that put you at risk, you still have to train intelligently. The freebie of a genetic advantage (such as some theoretical mutation that gives you Wolverine-like recovery powers) will run out eventually with age and cumulative stress.
- Pay attention to how well you recover from exercise. Look for how often you feel joint pain or other aches and pains, and how strongly. These may be related to your genetic makeup; they may be related to other environmental factors. Regardless, you still have to address them. If you’re always dealing with some minor problem, consider addressing your recovery more aggressively. You may not be recovering as well as you could be, or you may be following inappropriate training methods for your body.
- Time is a thing. Tissues remodel on their own schedule, no matter what you want. Good nutrition and regular movement help (by getting blood flow to the tissues as well as a mechanical signal to kick-start remodeling). Yet aside from “aggressive supplementation” with illegal drugs, we can’t do much about our natural tendencies. Give your tissues the time they need to build a strong foundation, and recover from training.
- Follow YOUR body’s cues, and don’t try to stick to a rigid workout schedule, or someone else’s workout plan. It might be too much work for you, or not enough. Schedules and plans are only as good as the bodies that can manage them.
Does exercise help you lose weight easily?
As we’ve seen, exercise and daily-life movement are part of a healthy lifestyle plan for everyone.
Exercise does many good things: improves our fitness and strength; build lean mass (muscle, bone, connective tissues); helps us beat stress; and so forth.
But does exercise always help us lose weight easily?
Many people who want to be smaller or leaner find themselves frustrated when they hit the gym regularly, and don’t seem to make much progress.
This may be due to poor training practices (in fact, it often is).
It may be due to other factors, such as not addressing energy balance.
It may also, in part, be influenced by genetic factors.
The FTO gene seems to be involved in various aspects of energy balance regulation, body size and fatness, along with other possible functions. For instance, recent research in mice suggests that it also contributes to skeletal muscle differentiation. (For more on FTO, see Chapter 7.)
One SNP on the gene is associated with both a higher BMI, and seeing different benefits from exercise, at least among people of European descent.
In one large study of several thousand people:
- People with the AA variant at rs9939609 tended to have a higher BMI (in other words, they were heavier), but also lost weight more readily when they exercised.
- People with the TT variant tended to be lighter, but lost less weight when they exercised.
But wait: There’s a wrinkle.
In the study used to support this finding, the effect was seen in people living in North America, but not in Europe. This may be because on average, Europeans tend to get more daily-life physical activity, such as walking or cycling, than North Americans.
In other words:
People with the same ancestry and genetic variant see different effects depending on their environment and choices.
At first glance, you might think this research says that exercise isn’t as helpful for weight loss people with a TT variant, and some people shouldn’t bother.
In fact, it suggests that exercise is more important, and more helpful, for people who might be genetically predisposed to being heavier.
In our experience coaching over 45,000 clients, we’ve found that the simplest explanations are often the most common.
While exercise alone doesn’t necessarily help people lose weight (if that’s their goal), exercise plus a few basic nutrition and behavioral habits, done consistently, does.
In other words, no matter what your genetic makeup involves, if you want to lose weight, the pathway to get there is still the same as everyone else’s.
Of course, exercise isn’t just about losing weight.
Indeed, there are two key problems with predictions about “exercise” and “weight loss”:
- There are many ways to exercise. “Exercise” can be all kinds things, ranging from yoga to extreme sports. Different types of exercise will affect our bodies differently.
- There are many reasons to exercise, such as having better metabolic health, better movement quality, less pain, more physical capacity, more strength, more muscle and bone mass, better athletic performance, and so forth.
Weight loss is just one of many possible effects of exercise, but certainly not an inevitable one, regardless of our genetic makeup.
What we found in our sample
In our PN sample, this FTO variant seemed to have little relationship to people’s response to exercise.
Only about 22% of our sample matched their predicted FTO profile; about 78% did not.
People who “should” have lost weight quickly didn’t (or perhaps even put on mass when exercising); people who “shouldn’t” have lost weight quickly did.
So, at least in our sample… FTO’s ability to predict weight loss from exercise… meh.
What this means for you
- You might find that exercise helps you lose weight quickly… or moderately… or not so much. This might mean you need to adjust your workout plan, be more consistent with exercise, or simply enjoy the health and stress-busting benefits of movement while looking for other ways to keep your body fat and body weight so that it’s in a healthy range.
- Movement is important for all of us, regardless of whether we are “genetically optimized” to benefit from it, or whether it helps us lose weight. (More on this in the next chapter.)
What’s next: Real-world strategies
By now, you may be wondering what you can do about your genetic makeup, whether you know you have risk factors or advantages.
In the next chapter, we’ll look at all the things that you can do to give yourself the best chance of living a healthy, happy, functional life… no matter what your genetic code is.